How do black hole jets form? Powerful magnetic fields launch electrically charged particles from the accumulated accretion disc material.
To help you wrap your head around this cosmic phenomenon, let’s take a ride through 7 key topics related to black holes. This way, we can piece together the known components in a comprehensive, yet easy-to-understand manner. Then, you’ll have a clearer picture of how those incredible black hole jets come to be.
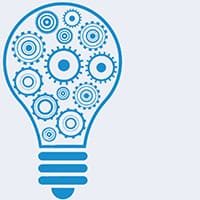
Important Note: My explanations are intentionally simplified. Even experts are still theorizing about many aspects of black holes. There’s so much we don’t know, and my shared points are a mix of theory, my own thoughts, and plenty of generalizations. Plus, I’ll be leaving out some complexities rooted in general relativity.
What’s more, our current “absolute truths” may turn out to be completely wrong. We need to keep pushing the boundaries of our understanding of physics to truly grasp the enigma that is black holes.
#1 What’s the Blandford Znajek process?
This is a way to extract energy from a spinning black hole. To make it work, we need two key ingredients:
- A rotating black hole
- A disc of plasma surrounding the black hole
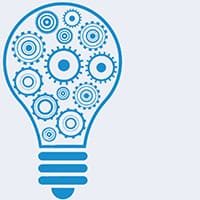
Important Note: When atoms zoom around like crazy, they can knock electrons off each other, creating ionized atoms missing one or more electrons. The remaining electrons and atomic nuclei make up plasma—an electrically charged gas that’s easily influenced by electric and magnetic fields.
#2 What’s a black hole?
Picture a region of space where the curvature is so intense that nothing can escape it—not even light. Mind-boggling, huh?
To get our heads around this, let’s tap into Einstein’s wisdom. His general theory of relativity portrays gravity as the curvature of spacetime. This means that anything with mass will bend the four-dimensional cosmic fabric.
Sure, it sounds like something from a sci-fi flick, but we’ve already verified some of Einstein’s predictions. For instance, gravity is an attractive force between masses, but it doesn’t directly affect light, which is massless. So, how does light bend around massive objects? The answer: spacetime distortion. We now understand that mass warps spacetime, and in the case of supermassive black holes, spacetime is so twisted that light can’t escape.
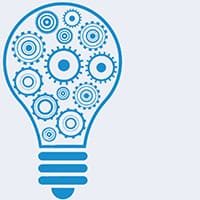
Important Note: Gravity doesn’t make massive objects interact with light. Instead, gravity makes massive objects interact with space, which then interacts with light.
#3 What’s the event horizon of a black hole?
This is where things get really intense in a black hole. Once anything crosses the event horizon, it’s headed straight for a singularity. That means there’s no turning back, and escape is impossible—even for light.
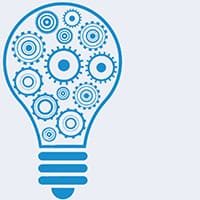
Important Note: A singularity is a region of space with infinite curvature. At the heart of a black hole, it’s the center point containing all the black hole’s mass in an infinitely small space.
#4 What’s a black hole’s accretion disc?
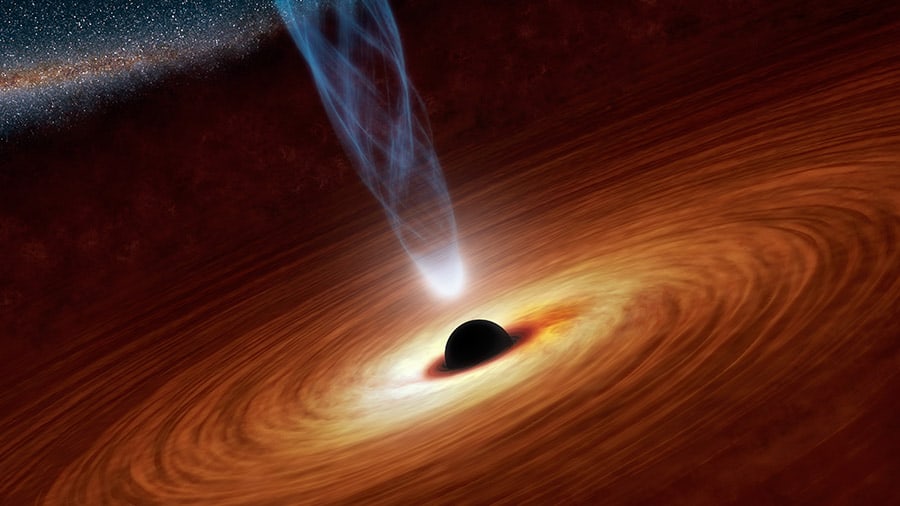
Accretion discs are these swirling masses of stuff – gas, dust, plasma, and other stellar debris – that orbit objects with super-strong gravity. They’re made up of protons, electrons, and radiation.
Picture this: a star orbiting a black hole, right? The black hole starts to suck material from the star, and that’s how most accretion discs form.
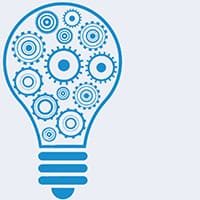
Important Note: Accretion disc material can heat up to thousands of degrees, and right before it hits the event horizon, it produces X-rays. For larger black holes, temperatures can even soar to millions of degrees, releasing gamma rays. This radiation is one of the ways we detect black holes.
So how come the material doesn’t just fall straight into a black hole, despite its powerful gravitational pull? It’s all about that angular momentum. Picture the International Space Station (ISS) zooming around Earth at 17,150 mph to avoid falling out of orbit. It’s a similar situation with accretion material and black holes.
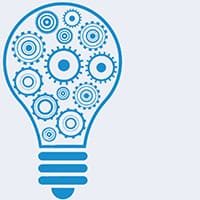
Important Note: If Earth stopped moving, it’d tumble into the sun. The Sun’s gravity would yank it in. But, Earth zips sideways relative to the Sun at around 2 miles/second. This is fast enough for Earth to avoid a fiery embrace with the Sun, but not too speedy to break free from the Sun’s gravity and leave the solar system.
Think of it like tetherball. Imagine the post is the Sun and the ball is the Earth, with the rope connecting the two representing the force of gravity. When you whack the ball, it spins around the post. If there’s no air or rope friction, the ball would spin around the post forever. This is how Earth orbits the Sun in the vacuum of space.
#5 How does accretion material fall into a black hole?
As we’ve seen, accretion material wants to hang out in orbit around a black hole. The angular momentum resists the collapse of material beyond the event horizon.
Over time, though, more material piles up in the accretion disk. Since materials at different radii orbit at varying speeds, atoms inevitably collide, creating friction and heat.
These collisions make the material lose angular momentum. The mass then moves inward and atoms lose electrons, becoming ions.
At the same time, magnetic fields form within the accretion disk from the charged particles. These magnetic fields then spin along with the black hole, while induced electric fields speed up the charged particles.
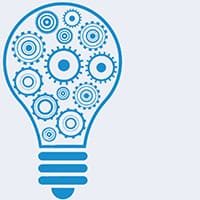
Important Note: A magnetic field forms when electric charges move. In the accretion disk, the charges rotate with the black hole. As a result, black holes become more magnetically charged over time as more material builds up in the accretion disk.
A changing magnetic field then creates an electric field. This is a cyclic feedback process, where one field constantly creates the other, resulting in an electromagnetic field.
All these forces make matter flow in complex, unpredictable ways. In turn, the material falls closer and closer to the black hole, while near the event horizon, the material speeds up.
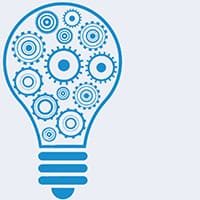
Important Note: Material near massive objects travels faster to maintain their orbit. That’s why satellites closer to Earth travel faster to stay in orbit.
Satellite altitude Satellite speed
Geostationary 35,780 km 11,100 km/hour
GPS 20,200 km 13,900 km/hour
Sun synchronous 705 km 27,500 km/hour
In this entire process, the density of the material increases near the event horizon. Here, atoms collide more violently, and the heating effect intensifies. Charged particles eventually tumble into the black hole, like a domino effect.
#6 How do black hole jets form from magnetic fields?
Finally, let’s dive deep into our main question.
To create these mind-blowing black hole jets, we need two things:
- Power source: to generate energy for the plasma
- Alignment: to keep the plasma flowing in a confined area
The power source is the rotating black hole. It spins accretion material at breakneck speeds near the speed of light. The material can heat up to several million degrees Celsius. This temperature spike happens in the inner accretion disk near the event horizon.
As we know, each zipping charged particle creates a magnetic field. These magnetic field lines weave through the entire accretion disc, getting even more intense near the event horizon. Here, the magnetic field lines violently reconnect and amplify due to a turbulent dynamo, a process where a spinning, convecting, and electrically conducting fluid keeps a magnetic field alive and kicking on an astronomical scale.
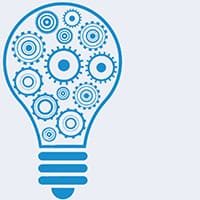
Important Note: There’s still so much we don’t know about the dynamo in accretion discs. It could be the result of different rotation speeds within the disc, or perhaps new poloidal flux merging with the original.
The same goes for Earth’s dynamo effect—we’re still figuring it out! We know there’s liquid separating the inner core and the mantle, which makes them spin at different rates. And as charged particles in the outer core move, they generate electric current, creating Earth’s magnetic field.
Now, picture this: a rotating black hole drags space along with it. It’s like the way water swirls when you flush a toilet.
This mind-bending effect of general relativity on spacetime is called frame-dragging. A black hole’s powerful gravity and rotational forces cause spacetime to rotate locally.
As a result, the magnetic field lines become denser and stronger closer to the event horizon. They end up looking like a tangled mess of wires.
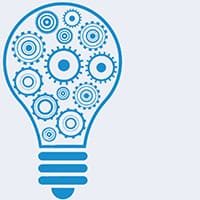
Important Note: Electromagnetic fields can’t escape black holes. However, when a black hole gobbles up a charged particle, its field line remains stuck to the black hole.
The flux conservation law tells us that field lines disconnected from their source can’t escape. These field lines get squeezed inward and forced to thread the black hole, held in the accretion disc by the Maxwell pressure of nearby field lines. If both ends of a magnetic field land inside the black hole, the field disappears. Picture magnetic field line B1 in the image below as an intact field line.
The magnetic field would vanish entirely in these scenarios:
- The accretion material vanishes, and charged particles cease to exist
- The accretion disc’s conductivity stops
Similarly, Mars might have once had a magnetic field. But since it couldn’t keep its core molten, its magnetic field disappeared.
Rotation of magnetic field lines
Spinning black holes drag the magnetic field lines of their accretion discs along for the ride. This rotation induces Electromagnetic Forces (EMF).
The magnetic field lines wrap around the accretion disc’s axis of rotation, creating a helical magnetic field in the Z direction. In this chaotic magnetic dance, the black hole devours much of the material, but some is catapulted into jets at nearly the speed of light.
This reveals the staggering strength of these magnetic fields, as it takes a colossal force to snatch charged particles from a black hole’s gravitational clutches.
Charged particles entering the magnetic field are then accelerated away in the jet. This launch direction is due to the fact that ionized matter can’t cross field lines because of the EMF. The mechanism is like a synchrotron.
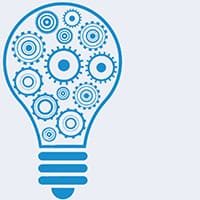
Important Note: Synchrotrons are massive machines that speed up charged particles. Picture these particles zooming along a fixed route, guided by magnetic fields like a roller coaster.
When objects don’t move in straight lines, they accelerate. That’s important because accelerating electrons give off radiation, like gamma rays.
Imagine plasma cruising along the magnetic field’s rotation axis. It’s like a synchrotron, where charged particles stick to paths shaped by helix-shaped magnetic field lines.
This is why black hole jets line up so perfectly. Plasma shoots out from both ends of a black hole while the spinning magnetic field transfers its rotational energy to the outflow. Let me break it down like an electrical circuit for you:
- The rotating black hole is like a battery (voltage source)
- Magnetic field lines act as wires
- Plasma is the current carried through the field lines
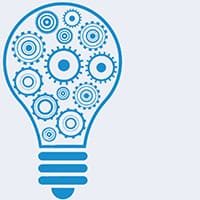
Important Note: Black holes lose some of their oomph during this process. They give away their rotational energy to the charged particles, which slows them down a bit.
#7 How much energy does black hole radiation release?
You won’t believe the crazy amount of energy black holes can produce! And nope, I’m not talking about what’s lurking inside the black hole itself. I’m talking about the stuff that’s being catapulted out from the accretion disc and the radiation it emits.
Before we dive into the nitty-gritty, just remember: mass is energy.
Using Einstein’s famous equation, , we know that energy equals mass times the speed of light squared. So, the conversion of matter into energy at a black hole’s accretion disc is just mind-blowing! It can reach up to 42%, which might not sound like much at first.
But, let me put this into perspective. Our Sun only converts a measly 0.7% of hydrogen mass into various wavelengths of light, with the remaining 99.3% becoming helium.
Think about this: our only way of converting matter to energy is nuclear power, where we convert just 0.1% of Uranium-235’s mass into energy. But if we could convert 1 kilogram of matter into 42% energy, we’d have over 10 terawatt-hours of energy—enough to power Earth for 30 minutes!
Check out the table below, comparing hydrogen fusion (which powers the Sun) to gasoline (which powers our cars). The 0.7% efficiency of hydrogen fusion is nothing compared to the 42% efficiency of black holes. Mind. Blown.
Fuel Source | Energy Density (MJ/kg) |
---|---|
Hydrogen fusion | 650,000,000 |
Uranium-235 | 88,000,000 |
Natural uranium | 81,000,000 |
Plutonium-238 | 15,000,000 |
Rocket chemical fuel | 50 |
Gasoline | 44 |
Coal | 24 |
Wood | 16 |
Lithium battery | 1 |
Capturing this incredible power is a whole other challenge. But who knows, maybe there’s an alien civilization out there that’s already figured out how to tap into this seemingly endless energy source…
“How do black hole jets form” wrap up
These amazing astrophysical phenomena aren’t just fascinating, they’re also potentially near-infinite energy sources. It would be like sci-fi on steroids if we could one day harness this cosmic power.
What do you think about how black hole jets form? Can we ever tap into black holes as an energy source?
Featured Image Photo Credit (cropped): NASA-JPL-Caltech
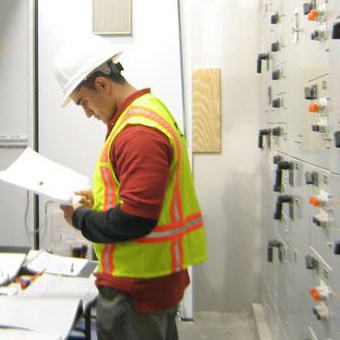
Author Bio: Koosha started Engineer Calcs in 2019 to help people better understand the engineering and construction industry, and to discuss various science and engineering-related topics to make people think. He has been working in the engineering and tech industry in California for well over 15 years now and is a licensed professional electrical engineer, and also has various entrepreneurial pursuits.
Koosha has an extensive background in the design and specification of electrical systems with areas of expertise including power generation, transmission, distribution, instrumentation and controls, and water distribution and pumping as well as alternative energy (wind, solar, geothermal, and storage).
Koosha is most interested in engineering innovations, the cosmos, sports, fitness, and our history and future.